Site-to-Site Process Transfer of a Liquid-Liquid Heterogenous Reaction- Replication of Mixing Environment to ensure Right First-time Transfer
Site to Site transfer of a process is a common occurrence in the industry. Unfortunately, it is rare for the reactors between the two sites to look identical and more common for the process to be transferred to a reactor / stirred tank that has a different configuration. If the product specs change during this transfer, this is more than likely caused by difference in mixing and/or heat transfer. In such instances, replicating the mixing environment between the reactors is extremely critical to maintain product quality attributes and achieving right first-time transfer.
This application brief details use of flow simulation using MixIT for ensuring same mixing environment from one manufacturing site to another for a liquid-liquid heterogeneous reaction. In this case, for a quick and successful transfer, suitability of a readily available reactor was evaluated in a systematic way using MixIT CFD simulations and modifications to the reactor to replicate process parameters and product specifications were quickly identified and implemented.
Site-to-site reactor comparison:
The reactor comparison is shown below for the transferring and receiving sites. Receiving site had a larger reactor. They planned for same occupancy and hence the batch size was 20% larger in the receiving site reactor. The transferring site reactor could be operated at 180 rpm. The receiving site reactor agitation speed was limited to 96 RPM for a routine gearbox setting and a maximum RPM of 150 was possible with change in gearbox setting.
Mixing parameters comparison:
Mixing in both the reactors was simulated and analyzed in MixIT. As the reaction is liquid-liquid heterogeneous, mass transfer coefficient KLa and hence interfacial area ‘a’ needs to be matched to ensure same process performance during the process transfer. In Liquid-Liquid systems, the droplet size distribution is a strong function of the shear rate generated in the reactor.
As seen from the simulation results in Figure 1, the shear stress generated at 96 rpm in the receiving reactor is much lower than the shear stress at 180 rpm in the transferring reactor. It was estimated that at 96 RPM the receiving site reactor can produce a dispersed phase droplets of average diameter of 309 m which is almost double the average droplet size produced at 180 RPM at the transferring site. To match the shear of the transferring site, the receiving site need agitation speed of 182 RPM which was beyond the motor-gearbox limit of available reactor.
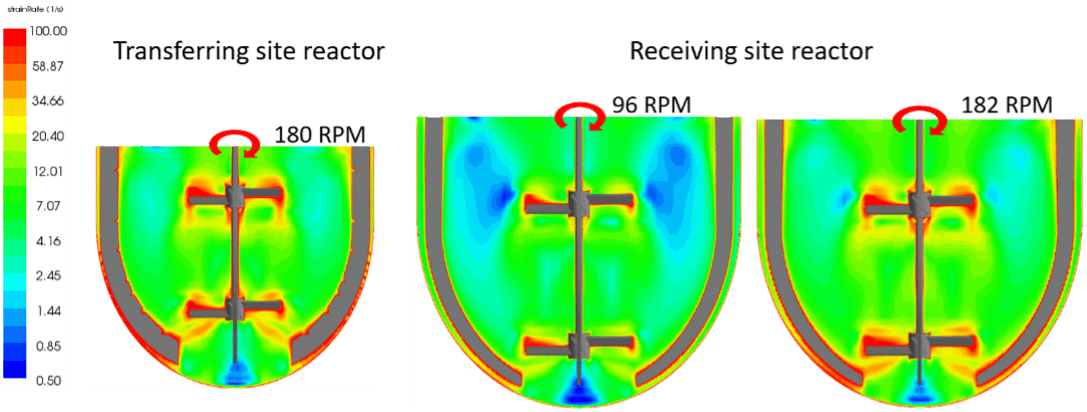
Figure 1. Comparison of Shear stress distribution between the transferring site reactor and the receiving site reactor. At 182 rpm, the receiving site reactor would generate similar droplet sizes as transferring site.
Two options were identified to resolve this issue. One is to reduce the batch size and the second would be to increase the agitator diameter. Both these options should help achieve the shear rates required.
Batch size reduction:
To match the mixing parameters of transferring site, it was decided to check batch size in the receiving site reactor which would provide required mixing parameters at 150 RPM agitation speed. Based on the power number available from above flow simulations and P/V calculation, a batch size with 515L total reaction mass was estimated and this was confirmed with MixIT CFD simulation.
A batch size reduction from 1365L to 515L, however, would have resulted in low throughput and longer delivery time impacting
business adversely. Hence, this was not viable.
Increase in the agitator sweep diameter:
Another way to match transferring site mixing parameters was to increase the sweep diameter of the agitator in the receiving reactor. The existing PBTD agitator had a sweep diameter (D) to reactor diameter (T) ratio of 32.6% and this could be increased to deliver more agitation power. A 20% increase in sweep diameter needs a PBTD with 600mm sweep diameter with D/T ratio of 39.1%.
MixIT CFD simulation with the new PBTD with 20% higher sweep diameter showed that 135RPM agitation speed is required to replicate transferring site mixing parameters. This was well within the existing motor-gear box setting delivering maximum agitation speed of 150 RPM. The new agitator blades were installed and the batches were executed successfully as per planned batch size and schedule.
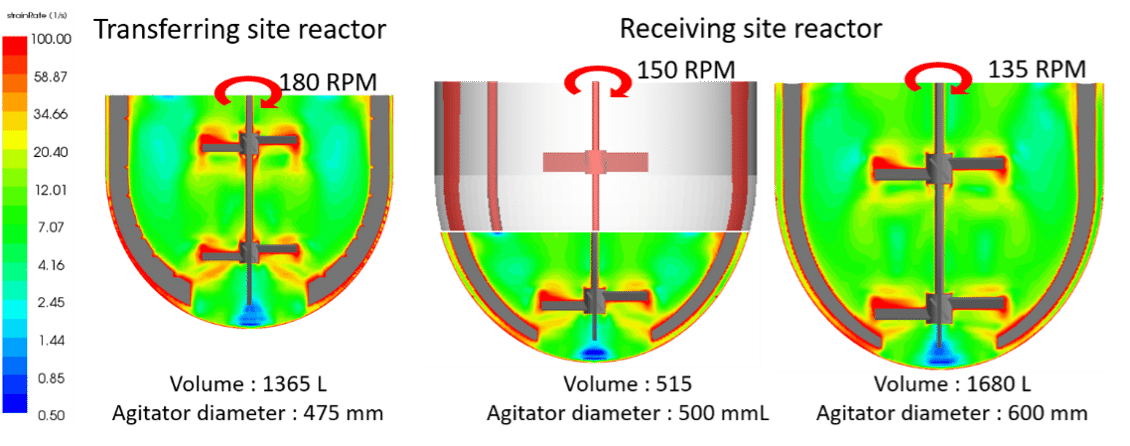
Figure 2. Shear stress distribution for reduced batch size (515 L) and for planned batch size with 20% bigger agitator. At both these conditions, the shear rates match the transferring site reactor.
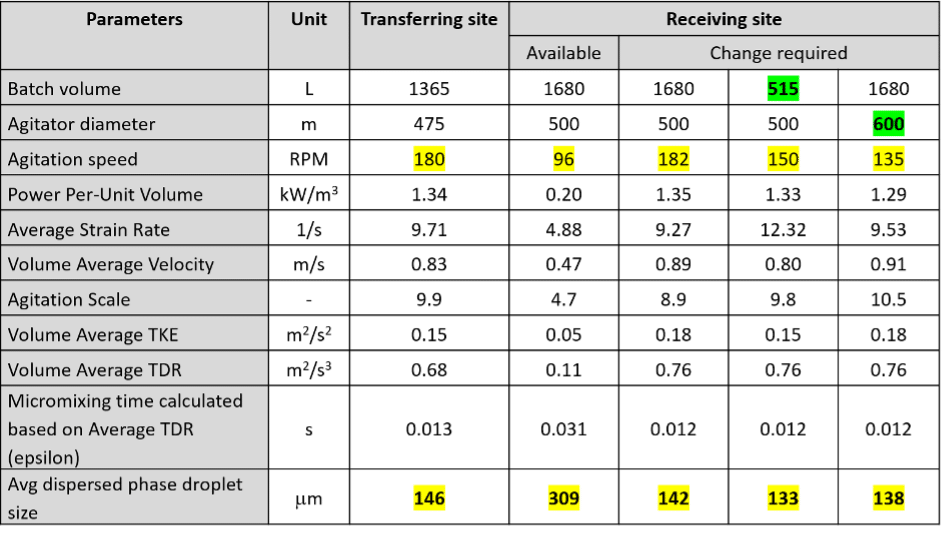
Table 1. Comparison of CFD simulation results for various design options for process transfer between the transferring and receiving site. Beyond P/V and tip speed, it is important to compare other mixing parameters. In this scenario, beyond mixing profiles the strain rates were compared to ensure droplet sizes were in the same range.
Conclusion:
MixIT CFD simulation allowed comparison of mixing parameters and in this case specially the average droplet size of the dispersed phase which directly impacts reaction rate kinetics for the liquid-liquid heterogeneous reaction being transferred from one site to another site.
MixIT CFD simulation allowed:
- Quick evaluation of available reactor suitability at the receiving site in comparison with transferring site
- Quick assessment for design changes which would allow execution of the process in the same receiving site reactor.
- A well-informed decision based on ‘a priori’ mixing analysis helped to evolve a simple and effective solution for site-to-site transfer
- Saved time, material and man power cost
- No batch failure
- Ensured timely delivery
Leave A Comment